France reshaped its military space structures in 2019, with the creation of Space Command (CDE, Commandement De l’Espace). This was due to a realization that although it was relying more and more on space capabilities, other countries were putting a lot of effort into defeating these assets. Consequently, France revised its space strategy, with a renewed emphasis put on Space Situational Awareness (SSA), which is the ability to know what is happening in orbit. This is critical in order to be able to detect any hostile act and attribute it to the right nation. So a good SSA system is necessary to deter other states from trying to sneakily attack French satellites. In order to do so, France plans to upgrade its Graves radar that keeps track of objects in Low Earth Orbit (LEO), and to increase it SSA in geostationary orbit (GEO) in several ways:
- First, by using networks of optical telescopes (called TAROT and Geotracker)
- Second, by putting intruder-detection cameras on its Syracuse IV communication satellites. These cameras will be derived from the star trackers carried by all satellites, but instead of stars they will detect moving objects that are coming close to the host satellite.
- Finally, by sending a pair of very small satellites with cameras to the geostationary belt, to be able to investigate suspicious activity from up close. This project, called YODA (Yeux en Orbite pour une Démonstration Agile, eYes on Orbit to Demonstrate Agility) will use platforms from French smallsat builder Hemeria, and should reach orbit by 2024-25. They will weigh 10 to 20kg each. In addition to SSA, it will help train space operators to operate and maneuver in GEO.
But all of this is not the active defense part. The ARES (Action et Résilience dans l’Espace) program calls for the deployment in 2030 of a very interesting satellite in GEO: a 100kg satellite with a high-power laser, as announced by the head of Space Command himself. The 2024-3030 military planning law even revealed the name of the weapon development program: FLAMHE. The use of a laser instead of a kinetic impactor comes from the fact that France has forbidden itself from creating dangerous debris that could damage other satellites. Laser, on the other hand, creates no debris, or only one if the target satellite is completely disabled. Ground-based lasers have already been used to blind observation satellites in LEO (and France has a program called BLOOMLASE to develop an operational capability), but the distance from the ground to GEO is roughly 60x greater, resulting in a reduction of received power at the satellite by a factor of 3600. Putting the laser on a satellite very close to the target makes for a much more workable proposition, and removes problems with weather, atmospheric turbulence, and thermal blooming. French company CILAS is working on high-power lasers, and has already demonstrated shooting down small drones with one, and has been asked to research a space version of this technology. The first mention of in-space use of high-power lasers by France was in the ground-laying July 25, 2019 Space Defense Policy speech by Minister Parly.
Laser system design
Using a laser could be extremely interesting to dazzle or damage hostile satellites in orbit, without producing any debris. The envisioned use probably looks like an hybrid between laser cutting and laser surgery: by targeting specific parts of the target with a high precision, it should be possible to punch holes in exposed sensors such as star trackers, in sensitive parts of payloads (for instance the pointing mechanism of reflectors for a communication satellite), or solar panels, in which individual panel section are usually connected by thin components.
There are two broad types of laser: continuous-wave lasers, which deliver a constant power over time, and pulsed lasers, which deliver all their energy in a very short pulse, but then have to wait for some time for the next pulse. They have different effects on the target material: with a pulse, the energy does not have time to spread by thermal conduction, so it can instantaneously vaporize a piece of the target if the beam is concentrated on a small enough surface. The vaporization can occurs so fast that is sends a shockwave into the target. This is a potentially useful side effect, but it can also result in an uncontrolled production of debris, which is precisely what the use of a laser was designed to avoid.

Continuous-wave lasers work a little differently. First, they have to heat up the target enough that it melts. For laser cutters on the ground, that is usually enough, as then the melted material can be blown away by a jet of compressed gas. Some lasers even blow oxygen on the area, which reacts with the metal and produces a lot of heat, which melts even more material. These two techniques a not likely to be used in space: first, blowing gas at the target requires to be extremely close (typically a few centimeters away in cutting lasers) and second it creates thrust away from the target, which has to be countered. Third, it also expends onboard resources, which limits the number of shots that can be fired.
So the laser will have to go beyond just melting the material. However, heating it up to vaporization requires a lot more energy, 25 times more in the case of aluminium for instance. Luckily, all the material does not have to be vaporized. If even a small fraction of it is, it will push away the rest (which has only melted), thus giving a clean cut. This makes the power requirement for a given cut hard to compute. Luckily, there is a handy calculator. For the spot size of the laser, which is limited by diffraction and depends on the size of the optic, the distance to the target and the wavelength, there is another calculator.

To get a sense of what can be achieved by a laser aboard a small 100kg satellite, let’s run some numbers. First, the satellite needs to generate power through it solar array. At the typical 30% efficiency, 1 square meter of solar panels generates 400W of electricity. Large GEO communication satellites can reach up to 20kW, but for our case 1kW is already a tall order.
Then, the fiber laser, like the ones used by CILAS for their HELMA-P 2kW anti-drone laser, have a typical electrical power to beam efficiency of 30% too. So the beam power would be 120 watts, with 280 of heat to be dissipated. HELMA-P is a continuous-wave laser apparently, but these figures would hold for a pulse laser too when averaging over time.
This power will be concentrated by the optic on a small spot on the target. Distance is a critical factor. 10m is too close for comfort, as the attacker might get hit by the target if it starts moving or rotating, and the solar panel wings of typical geostationary satellites extend to around 10m. So let’s assume the distance to the target is the next order of magnitude at 100m. Longer distances are also possible but spot area on the target depends on the square of the distance, so closer is generally better.

The next parameter to input is the size of the optic. The concepts of the patroller satellite show it is quite small compared to a communication satellite, and also quite flat, probably to make it easier to pack it as a rideshare for cheaper launch. So let’s say the optic has the diameter of a typical cubesat dimension, so 10cm. Then the calculator gives us a spot size of 1.3 mm diameter using a near infrared laser of 1064 nm wavelength. A visible laser would give a roughly twice smaller spot size. The depth of field, ie the range bracket at which the laser beam is smaller than twice the spot size, is 3 meters.
This small size allows to control very precisely control the effects on the target, by making very small holes and cuts. This avoids generating debris. Since the spot area is a square millimeter, concentrating a hundred Watts on it gives a power density of 10⁸ W/m², a hundred thousand times the power of the sun.
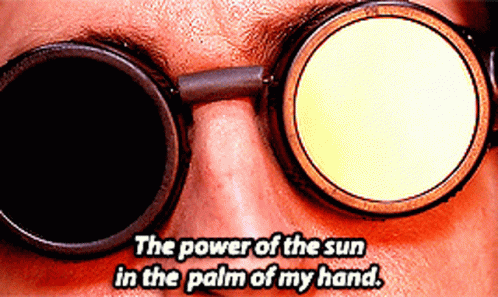
Making aluminium alloys (commonly used in satellites) melt when it is at room temperature requires roughly 1 MJ/kg, so with 100W that means it take 10 000s per kg of melted alumium, or 10s per gram. Volume-wise that means 30s for 1 cm³. Given the spot size of 1 mm², that means cutting through a 1 cm plate in 0.3s, and a cutting speed of 0.3 cm/s. All the computation above is a best case assuming no energy is wasted by having to bring some of the material to vaporization point, and not heat transfers in the material outside of the cut area, and that a 1 mm wide cut is wide enough not to have the metal seal itself back together (which should not happen, industrial cutting lasers have spot sizes of 0.1 mm or smaller, but that’s done in the atmosphere and with a jet of gas to get rid of the melted metal), and the material perfectly absorbs the laser’s energy. Still, in realistic conditions, the order of magnitude should be the same, and other materials will add variation in cutting time.
Fiber lasers have a mass of around 3 kg/kW for the laser source, so the laser subsystem of the satellite would fit easily in the 100kg mass budget. The mass of the focusing optics has to be added, though this optic might also be used for high-resolution or long-distance imaging of targets, so it is not only attributable to the laser. One thing to note is that the power level of the laser is low enough that only one fiber is needed and there is no need to combine several. High spectral purity fibers can reach up to 2kW, largely above the 100W considered here. Since there is no need for combination, lower purity fibers can even be used, and reach up to 20kW. They have the advantage of being more compact. If needed, even with only 1 m² of solar panels, a higher peak power of the laser can be reached by storing energy in a battery. After each shot, the battery is recharged, and the waste heat is dissipated. In the end, waste heat management might prove to be the limiting factor. Radiators are not shown on the depictions of the patroller, but are likely.

Overall patroller design
The laser might be the most striking feature of the satellite, but it is not the only payload. If everything goes right, it will never be used. What will be used is the rest of the payload, which will likely include imagers to detect, identify and characterize other satellites. For detection, the patroller will probably be cued by other assets, but since its main concept of operation is proximity operations in the geostationary belt, it will need to get a precise fix on its target once it is close enough. For this, a wide-angle imager is the best choice, and can be derived from star trackers (like the Auriga produced by French company Sodern, which is also building the intruder detection cameras on the Syracuse 4 satellites). It can also pull double duty as an actual star tracker.
One interesting feature in all the depictions of the patroller is that it has a set of two cameras pointed in the same direction. The cameras are quite far apart on the satellite. This points to a system used for stereo vision. By comparing the images produced by the two cameras, it is possible to estimate the range of the objects. This is very useful to discriminate stars from neighbouring satellites, and in the proximity operations phase, enables much quicker and easier operations. There is no need to drift around the target to range it, it can be done instantly and passively, therefore not alerting it it is being approached.
After a suitable position around the target has been achieved, the next thing to come in play will be a high resolution imager to identify it and characterize it. This will be useful to attribute the object to the right nation, to estimate its capabilities, and to find vulnerable points to target with the laser if need be.

This is the main payload and the concept of operation as I see them. In addition to that, the patroller will need a way to get to the target. Electric propulsion is a good way to do that, since it is very efficient and leverages the relatively oversized solar panels used to power the laser too. The French industry has several companies specialized in electric propulsion, such as Thrustme which advertizes a NPT300 ion thruster that uses 400W of power to produce 10mN at 2800s Isp, which fits very well with the concept. With 20kg of propellant for a wet mass of 100kg, it would give a 6.1 km/s delta-v, leaving a very large budget of 3.7km/s for operations in the geo belt after a low-thrust transfer from GTO to GEO (which uses around 2.2-2.6 km/s).
Station-keeping in geo orbit requires 47 m/s per year, but the annual delta-v budget should be higher as the patroller will likely maneuver around quite a bit. For instance, moving to the GEO graveyard orbit, 350km above the main belt, and circularizing there requires 13 m/s. From there, the patroller will drift 4.5°/day compared to the main belt, and be able to change orbital slot and surveil the population of inactive and active satellites, completing a full scan in 80 days. A faster drift speed will require more delta-v. A drop to 35 000km circular orbit will allow for a scan in 32 days, at a cost of 29 m/s.
The geo belt is not the only region of interest for such a satellite. France is planning to send other copies of it to low earth orbit (LEO), where it could be watch over very important assets like the CSO optical spy satellites, and gather intelligence on the observation satellites of other countries. Besides, having the ability to disable satellites in LEO without creating debris and a zero cost per shot is a very interesting counter to the military smallsat constellations that are becoming commonplace.



XSS-11, an US inspector satellite launched to LEO in 2005
Conclusion
Even with the large military budget increase that the new military planning law provides, France still has to make choices and cannot afford to fund everything. That space-based “defensive” capabilites are among the highly funded priorities shows that the space domain is seen as becoming more and more contested, and that it becomes necessary to have a credible deterrence there, allowing for a non-escalatory response in kind if French space assets are attacked. The use of the laser removes debris from the equation, simplifying the strategic calculus and making the capability more credible.
Thanks to ToughSF for helping out with his expertise on lasers!
[…] France is building a laser-armed killer satellite. What could it look like? — Read on satelliteobservation.net/2023/04/09/the-laser-patroller-satellite-an-elegant-weapon-for-a-more-civil… […]
LikeLike
thank you for the article
LikeLike
[…] Even with the large military budget increase that the new military planning law provides, France still has to make choices and cannot afford to fund everything. That space-based “defensive” capabilites are among the highly funded priorities shows that the space domain is seen as becoming more and more contested, and that it becomes necessary to have a credible deterrence there, allowing for a non-escalatory response in kind if French space assets are attacked. The use of the laser removes debris from the equation, simplifying the strategic calculus and making the capability more credible. — Read on satelliteobservation.net/2023/04/09/the-laser-patroller-satellite-an-elegant-weapon-for-a-more-civil… […]
LikeLike
[…] satellites in low earth orbit, where it has observation satellites to protect. By 2030, a larger, laser-armed patroller called EGIDE will be […]
LikeLike
[…] to national security. Alongside this an on-orbit laser is being developed under the designation FLAMEHE and a ground-to-space laser system known as BLOOMLASE. France intends for both projects to be […]
LikeLike